The ripples produced by my love of small waves
This is a story about an article roughly four years in the making; about my fascination with how a tiny animal moves; and about the experimentation that was necessary before I could answer the questions that first piqued my interest.
Larvae of the fruit fly, Drosophila melanogaster, crawl forwards by means of waves of muscle contractions that travel from the back to the front of the animal. I find watching these waves of contractions mesmerizing. When I hook up my speaker to the recording equipment and listen to the rhythmic bursts of electrical activity underlying these waves, I find it soothing. I was intent on learning more about how the nervous system produces this activity.
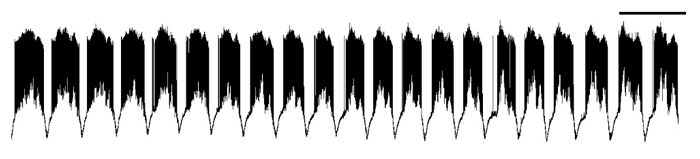
An example of the rhythmic electrical activity I so love watching and listening to! Each one of those black bursts represents muscle activity recorded during a single wave. These waves continue spontaneously for several minutes. Scale bar at top is 20 seconds.
Motor activity, like that underlying crawling, is generated by networks of neurons. At the last output level of the nervous system are the motor neurons, which send signals to the muscles, causing them to contract. Current research in motor control is focused on finding out how much motor neurons contribute to shaping the timing of motor behaviors. In other words, are motor neurons just passive relayers of the signals they receive? Or, do motor neurons shape the signals before passing them on to the muscles, thereby influencing the timing of the behavior? It is now well accepted that motor neurons have active membrane properties – there are ion channels in the cell membrane that allow motor neurons to respond to input in non-linear and diverse ways. However, few direct tests of the role of motor neuron active properties in shaping motor behavior have been carried out, and many questions remain about the role of specific ion channel genes in motor neurons. These are some of the questions I set out to answer. But first, I had to figure out how to record activity in animals that could fit several times over on my smallest fingernail.
Electrical activity is often recorded by inserting glass capillaries with small, sharp points into a cell. Inside the capillary is a conducting salt solution and a wire, which carries the electrical signals to an amplifier. Typically, all efforts are made to create stability in the experimental setup – animal preparations are pinned down to reduce movement; recording setups are placed on tables that float on air to reduce vibration. However, when recording motor activity, movement is inevitable. When I prepare a larva for recording, I cut up the middle and open it up like a book. It is pinned at the head, tail, and four corners, but the muscles are free to move. Waves of muscle contractions that would produce crawling if the larva were intact are still observed. This is great, because it is exactly this activity that I want to study, but it presents a problem for recording. Each time the muscles contract, they are displaced from their resting location. The displacements are large, at least relative to the overall size of the larva, and can dislodge or break electrodes. I cannot grab on to the muscle or restrict its movement in any way, since it would alter the activity. Eventually, I solved this problem by making special glass capillaries with very long tips. These tips were so long, in fact, that they were flexible despite being made of glass. In this way, the electrode tip moved with the muscle as it contracted and the recording could be maintained.
After solving the problem of recording stability, I realized there was another issue: muscle damage. The dorsal-most muscle I wanted to record from risked damage during the midline cut used in the classical dissection. So, I decided to make the cut to the right of the midline instead. This meant that the nerves running to some of the dorsal muscles on one side of the animal were cut. However, it left the muscles and nerves on the other side perfectly intact for recording. I was delighted to see that the waves of muscle contractions were still present. But I couldn't simply assume the timing of the activity would be the same as that seen with the classical dissection. Before proceeding with experimental manipulations, I needed to characterize the activity in control animals. I recorded the muscle activity of larvae dissected with the classical and new dissection methods. What I found was that the larvae dissected with the new method had activity that was similarly rhythmic and coordinated but was faster than the classically dissected larva. This result ended up going into the supplementary materials of the paper, since it was tangential to the main result presented. However, it was interesting because it suggested a role for sensory feedback in regulating the frequency of motor activity, something that has been seen in other motor systems.
Finally, I was ready to address my main research question: How does altering the expression of specific ion channel genes affect the timing of crawling-related activity? I focused on a gene called slowpoke, which encodes for a large-conductance calcium-dependent potassium channel. These channels are particularly interesting because, unlike many channels, they require two signals to maximally activate: a change in voltage and an increase in intracellular calcium. The gene was originally called slowpoke because mutating it in adult flies causes them to be lethargic and fly very little. I was curious to see what would happen to larval motor activity if I reduced slowpoke expression. I started by expressing a slowpoke mutation in the whole animal, and noticed that mutants were capable of generating the same waves of muscle contractions seen in controls. What was different, though, was the timing of the activity. The activity I recorded in mutant animals was faster than that recorded in controls, with decreases in the time a single muscle was active during a wave cycle (burst duration), the time a single muscle was inactive (quiescence interval), and the total time it took to complete a wave cycle (cycle duration). These larval slowpokes were quick!
One of the problems with whole-animal mutations is that they do not tell us a lot about which cells are responsible for the effects we see. I wanted to know which changes in the timing of the motor activity might be due to reduced slowpoke expression in motor neurons. So, I used special genetic tools to target a slowpoke manipulation to select, identified motor neurons. Since the neurons were not easy to access for recording, I recorded from their muscle targets as a proxy for their activity. The changes in the timing of the motor activity in manipulated animals were not as quantitatively large as in mutants but were qualitatively similar. Most importantly, while the burst duration was unchanged in manipulated animals, the quiescence interval between bursts was reduced relative to controls, leading to a decrease in the cycle duration. In other words, the motor activity was faster in manipulated animals, suggesting that motor neuron active properties play a role in regulating the frequency of motor activity.
Like many (and I would argue, good) research projects, my results led to more questions. Why did mutating slowpoke lead to opposite effects in larvae versus adults, speeding up motor activity in the former and inhibiting it in the latter? Which effects were due exclusively to the reduction in slowpoke expression, and which were due to compensatory changes that often occur in the nervous system when gene expression is manipulated? I didn't have all the answers. But I felt that apart from the original results presented, one of the strengths of the paper was that it identified holes in our knowledge and proposed future experiments to help fill those gaps. For example, expressing slowpoke manipulations at distinct time points in the animal's development could answer questions about the role of compensation in maintaining crucial motor behaviors. I suggested several others experiments in the discussion of the paper that I think could advance our understanding of motor control. I hope I, or other researchers, get the chance to tackle them soon.
The moment I submitted this manuscript was a beautiful one, made all the more so because I was able to do it in accordance with my views on the importance of open science. I uploaded a preprint to figshare for all to read and comment on, and just a few hours later, I submitted the manuscript to the open access journal, PeerJ. The rest, as they say, is history...which you can also read online, since the peer-review history is published alongside the article. Go, open science!

COMMENTS